Swords, Sports Cars and War Machines
- Asante Lawla
- Apr 23, 2023
- 15 min read
Updated: Sep 19, 2024
Muscle Power?
In traditional martial arts, we often talk about trying to reduce our reliance on muscular power and instead opt to use mass, relaxation and eventually elasticity when generating force. To some, this may sound almost counter-intuitive. Surely if you want to produce as much force as possible you want to recruit as many muscle fibres as possible, no? Well you’d think so, but the problem isn’t quite that simple.
Muscles have a built-in protection system called autogenic inhibition, which causes them to fail when they experience loads beyond a certain threshold and reduces neural drive to other muscles involved in the action. For example when you roll your ankle, your entire leg ceases to function momentarily. This helps to prevent muscle tears, but complicates the force production problem, especially when speed is a requirement. See, the faster a muscle contracts (concentrically), the less force it will produce because of the innate force-velocity relationship. I.e. You may squat 150kg, but can only do so slowly, as moving any faster reduces the time available for the requisite chemical reactions to take place.
To understand this problem properly, we have to keep in mind that many traditional arts evolved from combat on a battlefield. Scenarios involving heavy armaments, charging armies and massive impacts. This meant that the type and amount of force experienced in a typical collision would far exceed what you might expect in normal combat sport, having a drastic effect on muscle function. The Status Quo
Striking focused combat sports, typically involve knocking your opponent out or landing as many strikes as possible using the ample time and space available. As a result, athletes tend to favour a “stick and move” approach where striking technique minimises contact time. This allows fighters to reposition, score more points and reserve heavier strikes for opportune moments. In this context, autogenic inhibition doesn’t really pose much of a problem. With a "snappy" jab/cross, you can deliver an adequate amount of force to knock someone out, without risking muscle failure. However, in a battlefield context, the opposite is true. Often, warriors had limited time and space for each engagement, whilst their main objective was to repel/route the opposing army. This made things like “stopping power”, “creating space” and “follow through” to penetrate armour, all major priorities. Not to mention that these strikes were largely limited by muscles in the hands, gripping onto a weapon, lacking the luxury of ramming solid bones into someone. In the modern age these would all be described as kinds of “pushing strikes” which, although are used, tend to play second fiddle to a more “snappy” kind of striking. All in all, the elevated forces involved during clashes, and the change in striking priorities, makes autogenic inhibition and the inability to rely on muscle recruitment alone a very likely scenario.
Fortunately, the human body isn’t the only power delivery system which suffers from this problem. We can look at another system and how it overcomes the problem, to better understand how we can do the same.
The Combustion Engine
Like muscles, combustion engines in cars fail under excessive load. This is called stalling, and is actually an excellent analogy for autogenic inhibition.
One way engineers could solve the problem, would be to just increase the power output of the engine. That would allow a driver to ignore the stalling problem, but in exchange introduce a host of other problems, including; burning through fuel reserves too quickly, difficulty in controlling power output, and extra stress on engine components that could also lead to damage or failure.
The more efficient way of managing the problem is to introduce a “transmission” system alongside “launch control”, which is what we’ll be looking at in this article.
Transmission
If you’ve ever driven a manual car or rode a mountain bike, then you’re probably already very familiar with transmission systems and have some idea of how they work. But for the sake of clarity, I’m going to go through it in a little more detail just so that we’re all on the same page.
Transmission systems help you to manage load on the engine, and give you more control over the application of power output using torque conversion.
Torque Conversion and Engine Load
Engine load is the amount of work the engine has to do, relative to the amount of power it can produce. Using gears we can reduce the load by reducing the amount of work the engine carries out per revolution.
For example, the fuel in this engine produces just enough energy (E) to turn the wheel once per revolution.
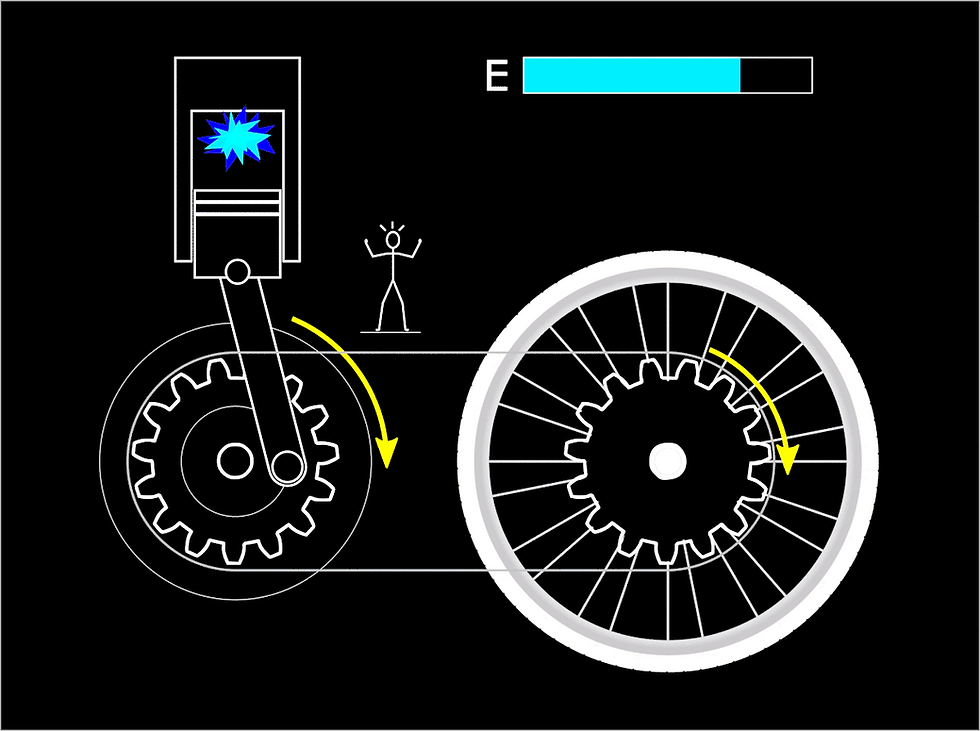
Fig 1
We can make life a little easier on the engine by switching to a lower gear, cutting the amount of work in half (Fig 2). Now the wheel will move at half its previous speed, but receive twice as much energy in each complete rotation. This is what we call a torque conversion. In exchange for speed we increase the amount of torque (rotational force created by the engine) the wheel receives as it rotates. Another way of thinking about this is increasing the energy density of the wheel’s movement.
Torque conversion is why machines like tractors, freight trains, and heavy goods vehicles move and accelerate slowly, but can pull very heavy loads. It’s also why we always select the lowest gear first when moving a manual car/bike, as our initial movement requires a lot of energy to overcome inertia.

Fig 2
So far so good, but what if we want the wheel to go faster to get more work done ‘quickly’, aka power?
Torque conversion also works in the other direction, allowing you to increase speed whilst 'lowering' the torque received by the wheel. This may sound impossible at first, but there is a trick to it. If we want to increase the work done per engine revolution, we have to figure out a way of doing so without causing the engine to stall. Remember the engine produces a finite amount of torque. We can’t pull energy out of thin air, but we can take advantage of momentum (P), which is the next best thing.
By letting the wheel build up rotational momentum before changing gears, we can reduce load on the engine using the wheel’s momentum as an additional energy supply. We essentially turn the wheel into a battery that stores energy for later use. In cars we also have a “flywheel” attached to one end of the engine, allowing it to store rotational energy, even when the car isn’t moving. With this method we can get the wheel to spin twice as fast as our simple engine (fig3), despite the engine producing the same amount of torque. This is why sports cars produce far less torque than HGV’s, but travel much faster using aggressive overdrive gearing design.
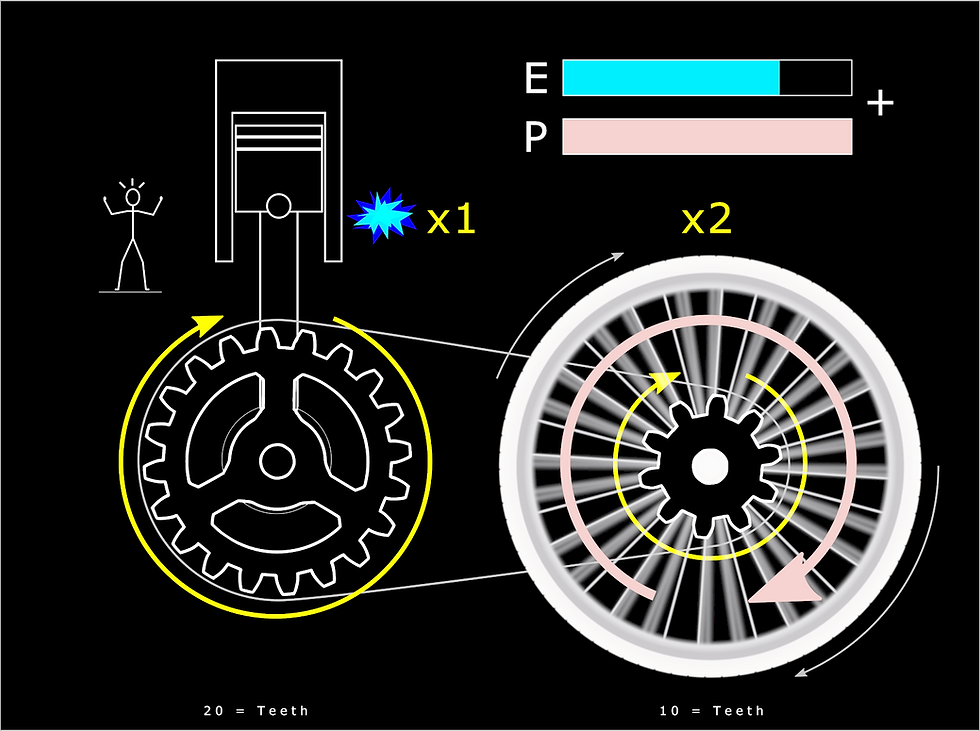
Fig 3
So far we’ve learned that torque conversion allows us to reduce speed in exchange for more energy when carrying out heavy tasks, and that we can use momentum as an additional energy source. But how does any of this relate to swordsmanship ?
In Shastar Vidiya for example we use a method of draw cutting that prioritises stopping/holding power. In the context of a battlefield, it’s important we have enough energy to; maintain pressure throughout the draw, prevent our weapon from getting stuck, and effectively stop charging opponents. For these reasons we use something called “soft heavy power”. Written another way, a movement that starts slowly (relative to our body) but feels incredibly heavy, then increases in speed and power as it continues. Much like what we’ve described above. The only difference being, the human body doesn’t have convenient gears it can switch between. That said, it can go one step further by making use of another clever trick involving momentum called “momentum transfer”.
Momentum Transfer
Momentum transfer is when momentum from one object moves into another object. If you’ve ever played football, bowling, baseball or any sport that involves accelerating a ball, then you’re probably already very familiar with the physics of it. You probably already know that the more momentum (linear or rotational) you generate before you hit/throw a ball, the faster that ball will go. This is why we instinctively take a “run-up” or “wind-up” when we want to make the ball go further. What specifically interests us however, is how momentum is transferred internally to significantly increase available energy, as well as how our muscles/connective tissues affect this transfer.
“Whiplash” is a great example of this phenomenon. Studies show that during a car crash, even at a low speed of just 8mph, your head can experience anywhere between 3.5 G’s and 7 G’s of acceleration. At he top end, that’s double what an astronaut experiences during a rocket launch! Undoubtedly outside the acceleration cervical flexors (neck muscles), or even cars for that matter are capable of producing. This is only possible because of an energy increase that occurs during momentum transfer. Remember, energy can neither be created nor destroyed, and momentum in an closed system is always conserved. Following these laws of physics, during whiplash, when the seatbelt causes the body to stop moving, its momentum and thus energy must be transferred to the head (fig 4). Note, it requires a lot of energy to keep a heavy object in motion. If you take that energy, and transfer it to a much lighter object, its energy and thus speed will increase considerably. Also if we’re talking about getting work done (like draw cutting for example), the lighter object will now have far more available energy to finish the job, even if it experiences heavy resistance.
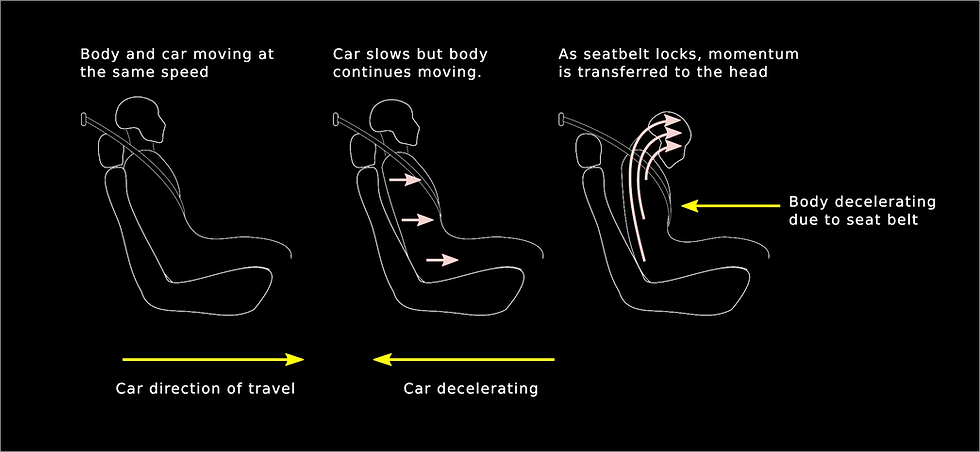
Fig 4
So all we have to do to overcome the muscle failure problem is tap into our body’s momentum and initiate a transfer from our body to our weapon. Problem solved!!… sort of. There are still a few more things we have to think about.
As I said before, none of this is new knowledge. We all know that you have to take a run-up / wind-up to hit harder, but that isn’t always possible or even practical on a battlefield. How would you pull that off whilst holding a line? Do you really want to take a massive wind-up, telegraphing your movement before striking? You can see how these methods may be problematic. So how does what we do in Shastar Vidiya differ from the norm?
To explore this, let’s look at another practice which makes use of momentum transfer, like the popular sport of Olympic javelin throwing and compare.
In a similar manner to whiplash, javelin throwers initiate a momentum transfer by taking a run up before digging their heels in and bringing the body to an abrupt halt. This causes a transfer of momentum from the body into the javelin, but relies heavily upon ground conditions, muscular contractions and footwear.

On a battlefield, good ground conditions are anything but guaranteed, meaning the javelin method would more likely result in sliding. Ancient warriors were expected to fight on unconsolidated, uneven, and possibly even waterlogged ground (India has a very heavy rainy season). Quite unlike the specially designed Tartan Track used in Olympic stadiums, that makes the javelin technique possible. This aptly brings us to the topic of launch control.
Launch control
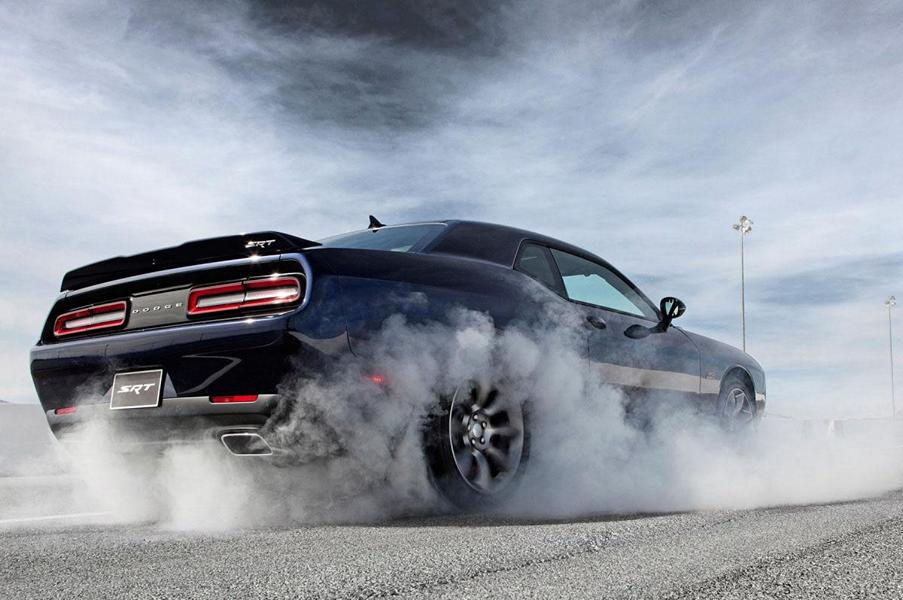
Ancient warriors were not the only ones who had to contend with adverse weather and ground conditions. Racing drivers also had this problem which is why traction and launch control were introduced. Launch control is used alongside a transmission system to ensure the application of force on the ground and thus loads on the engine are applied smoothly. This helps to prevent stalling / engine damage and reduces wheel spinning where energy is absorbed by the ground. We want to do the same thing when striking to avoid autogenic inhibition and ensure an efficient transfer of kinetic energy to our weapon and the opponent's body.
You can visualise the GIFs below as a simplified version of the engine, where load takes the form of a steep hill.
A sudden load can cause the engine to stall, or damage its internal components, as its kinetic energy and momentum are rapidly drained.
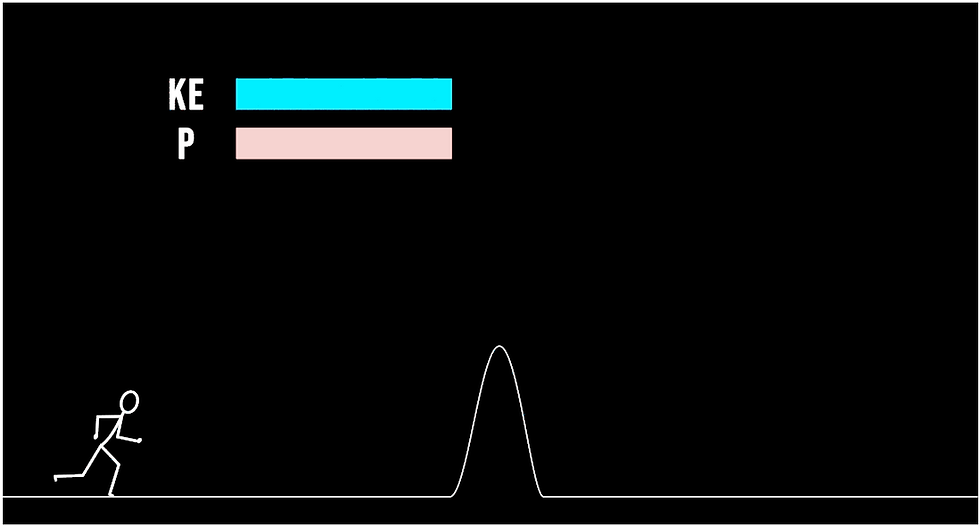
Fig 5
Whereas if we take the same load and apply it gradually, energy and momentum are better conserved, making this type of application much more efficient. This is essentially what launch control achieves for cars. It ensures load is applied smoothly to reduce energy loss.
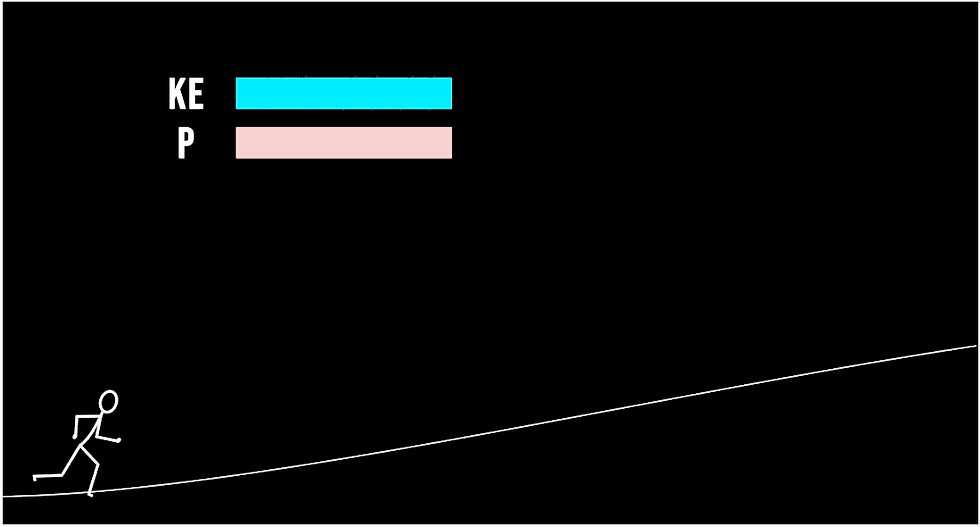
Fig 6
For a javelin thrower launch control isn’t so much of an issue. Their application is a lightweight javelin, and the ground that they run on is specially designed to allow them to stop quickly. Whether they stumble or skid after they’ve thrown the javelin, isn’t important as long as they stay within the throwing area. Because of this they can opt to use powerful leg muscles to bring the body to a sudden halt. It goes without saying that skidding or stumbling isn't something you'd want to experience on a battlefield, but the more subtle downside to this technique is the loss of energy due to absorption via muscle tension.
When a javelin thrower stops, their legs and core muscles behave a bit like shock absorbers, absorbing / cancelling out some of the energy that could be transferred to the javelin (fig 7). Though the amount is negligible is this application due to the athletes initial speed, the principle is carried across all movement. The more isolated the muscular tension you have in your body, the greater the impedance to the flow of momentum.
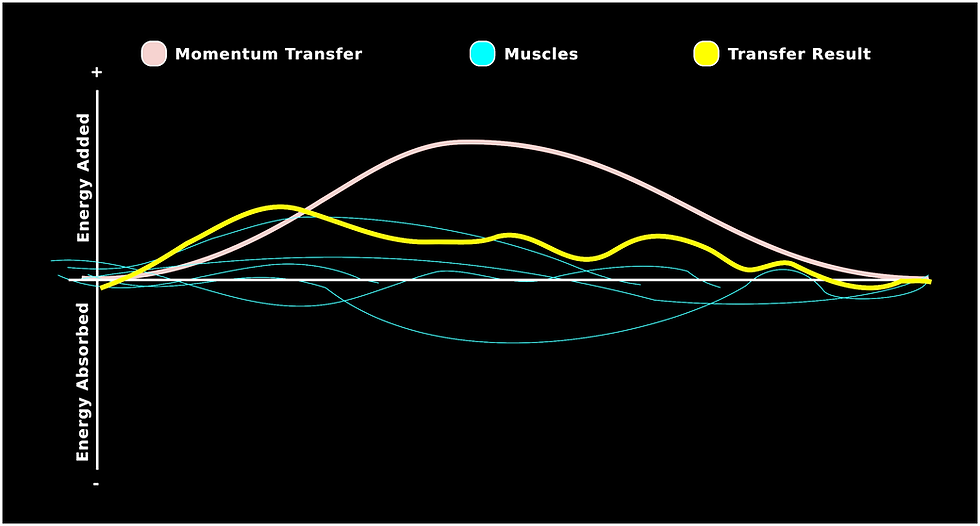
Fig 7 (Over exaggerated for clarity)
You may think, “but can’t they just limit tension to only the muscles that will work with the momentum transfer, rather than against it?” In an ideal world, yes, but in reality, producing enough force to bring the body to a sudden halt whilst doing so, is extremely difficult. This is especially true when using core torsional strength which requires you engage a whole host of muscles for stability, alongside the muscles doing the primary work. Furthermore, tight muscles are closer to their failure threshold and they’ll receive more shock than surrounding more relaxed muscles, making them more prone to injury.
So how do we initiate momentum transfer with some form of “launch control”?
Despite our intuitions, transferring momentum isn’t strictly about speed, it’s about creating a change in momentum. Going from a sprint to a sudden stop is only one way of creating a large change. Momentum, being a vector (direction) as well as a magnitude (amount), means we can also create a large change by simply changing direction. For example, going from 20mph to 0mph in under a second, is similar to going 10mph one way, then 10mph the other way, in under a second. Understanding this means we can move slower and thus in a more controlled manner, whilst transferring the same amount of energy.
That leads us back to where we began this article at the importance of relaxation and controlling our mass in SV. Over the years Gurudev Nidar Singh has used various phrases to try to describe this, such as “go backwards to go forwards” or “turn circles into straight lines and straight lines into circles”. But my favourite analogy of them all and the one I happen to think is the most practical is to “move like a trebuchet”. This constitutes our base method for generating force.
The Trebuchet.
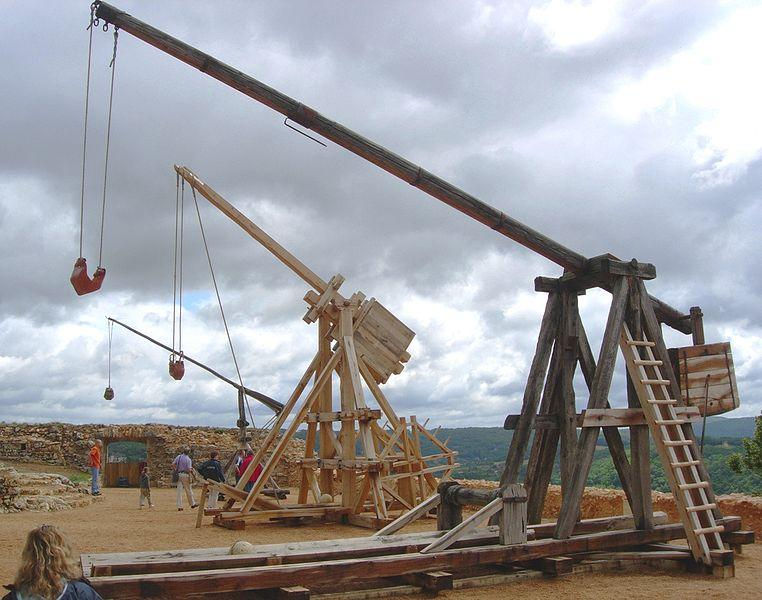
Way back before cannons and gunpowder were readily available, armies would use various types of catapults to launch projectiles at each other. One of the most famous and powerful versions of these weapons was the trebuchet, capable of throwing a 90-130kg payload up to 300m. Although this may not sound like much by today’s standards, when you consider that this was achieved without explosives and managed to outperform machines using incredibly tensioned sinew and wood (coincidentally quite analogues to muscles and bone). The ingenuity behind the design becomes more apparent.
Unlike the popular ballista, which fired relatively light loads, and the mangonel which were a pain to reload. The trebuchet had few caveats, able to turn a low velocity, relatively low stress movement, into the most powerful catapult in history. These advantages carry over to the human body, and as you may have already guessed, are achieved using yet another energy transfer technique. This time relying on gravity.
Just a note, we’ll be using a slightly modern take on the trebuchet that has wheels attached. This feature is important for understanding how we move without relying too much on the ground.
If you’ve understood everything up until this point, then understanding the trebuchet is fairly straightforward. In the picture below you’ll notice that the tip of the trebuchet will move much further and thus faster than the counterweight as it rotates (fig 9). This changes the torque output much like when you shift up a gear in a car. Also because the tip of the trebuchet is constantly changing direction, (moving in a curved line), you have a constant change in momentum transferring energy to the payload, also known as a fake “centrifugal force”.
Generating the energy for this movement is a counterweight raised above the ground which when released, converts gravitational potential energy (GPE) to kinetic energy.
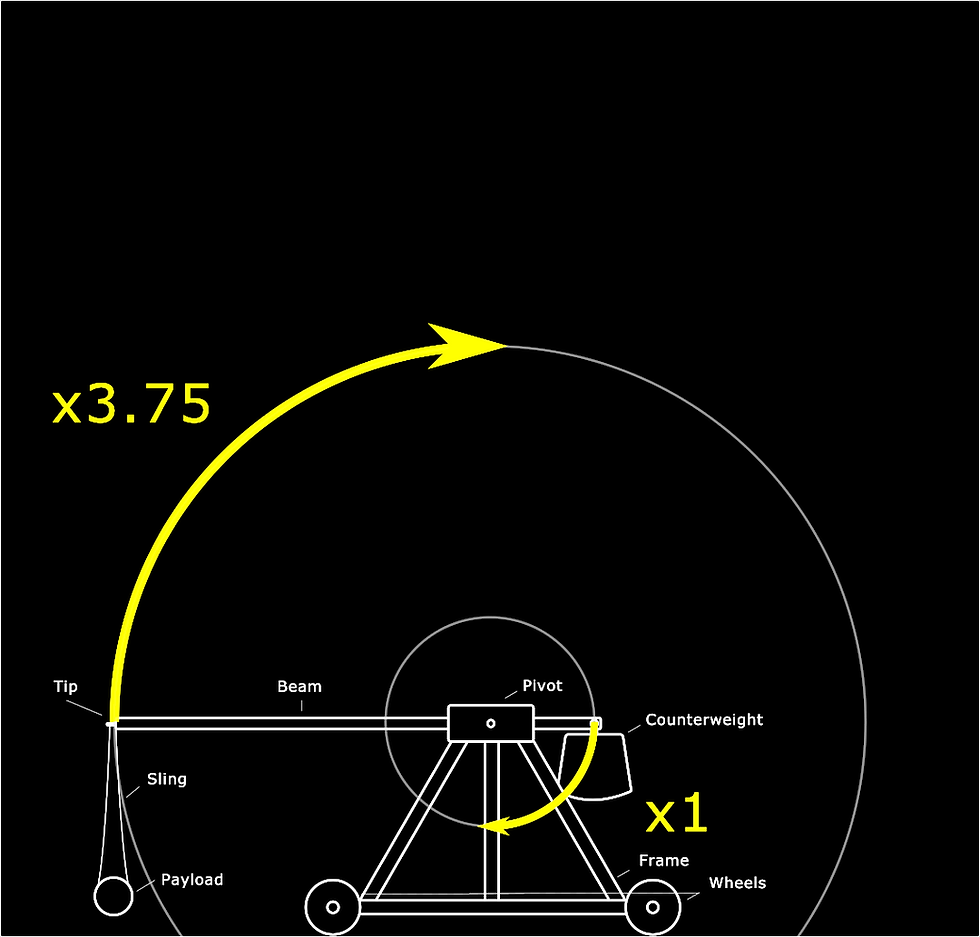
Fig 8
As the counterweight is released, the entire frame of the trebuchet will roll forwards on its wheels. This is a result of gravity pulling the weight directly downwards and the force required to change that direction, being greater than the force needed to overcome the frame’s inertia and cause it to roll forwards. Then, when the counterweight approaches its lowest point, it will momentarily slow before changing direction to swing horizontally. As previously discussed, since energy cannot be destroyed, most of its kinetic energy is transferred through the path of least resistance to the payload via the beam and sling. Finally, the sling increases the payload's speed and facilitates an efficient transfer due to it being lightweight and supple. I.e. Chains are easier to move than steel rods because they can be moved in parts rather than as a whole.
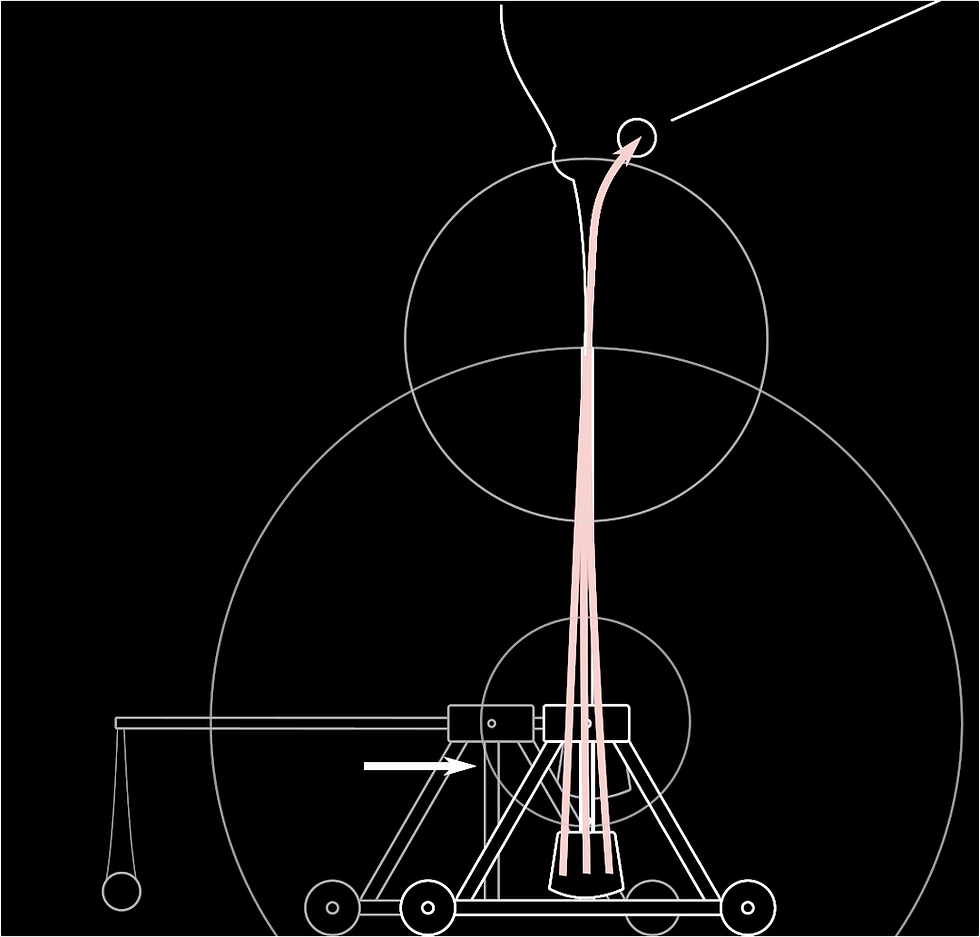
Fig 9
To help with visualising this, here’s a quick physics simulation comparing different trebuchet mechanisms. Watching closely you can see the exact moment the counterweights slow and compare how the sharpness of the change in direction increases the payload speed.
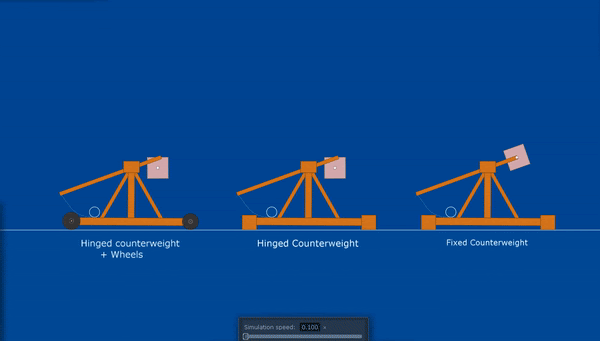
(Turning a circle into a straight line and a straight line into a circle)
Applying this to the human body, when we relax through a movement and allow ourselves to “sink”, we’re essentially releasing our body mass from its constraints, allowing it to act a bit like the counterweight in the trebuchet, providing we do so in the correct manner.
Below is another simulation showing energy being transferred from the body into a ball, via the arm. It provides a comparison between using tension to initiate the transfer, a theoretical hard stop and adding gravity into the mix.
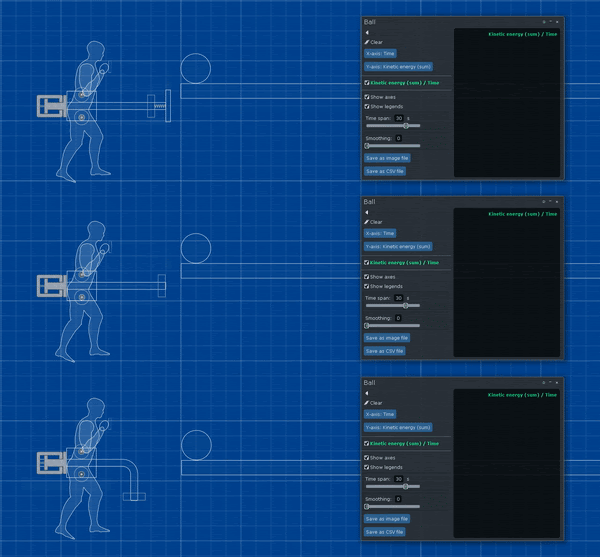
The top method uses tension in a spring to simulate the use of muscle to halt the body Energy transferred - 3.5J
The middle method is a theoretical instant stop, using a simple block Energy transferred - 4.5J
The last method uses gravity and rail to change direction smoothly. Energy transferred - 7J 100% increase over the spring method
(Double that of the spring method !)
The “Internal” Effect
This will be covered in more detail in another article, looking at other mechanisms of muscle function, primarily, alpha-gamma coactivation vs the myotatic reflex. In its entirety, and when accessed via the subconscious this forms the basis of a whole separate skill related to developing power.
There is also a more subtle internal effect as our "released mass" pulls on and stretches connective tissues throughout the body. You’ve probably felt this effect numerous times throughout your life but never noticed. A typical example would be when carrying something really heavy in one hand, causes the opposite arm to lift up involuntarily. The weight of the object literally pulls on connective tissues across the shoulders creating a path of least resistance for energy to travel through and raise the arm.
We can use this to not only transfer energy more efficiently, but also to aid in forming structures which reinforce our movements and better coordinate our muscles when they do need to contract. The stretching of our muscle fibres increases their sensitivity to changes in load and improves interconnectivity, which in turn; better distributes load, improves reaction time and synchronicity, further reducing the likelihood of muscle strain leading to a failure cascade.
The simulation below shows the difference between an individual muscle contracting alone, 2 muscles of equal power working together, and finally the effect of mass being “released” to tauten connective tissues internally, acting a bit like the counterweight in the trebuchet to transfer GPE to the ball.
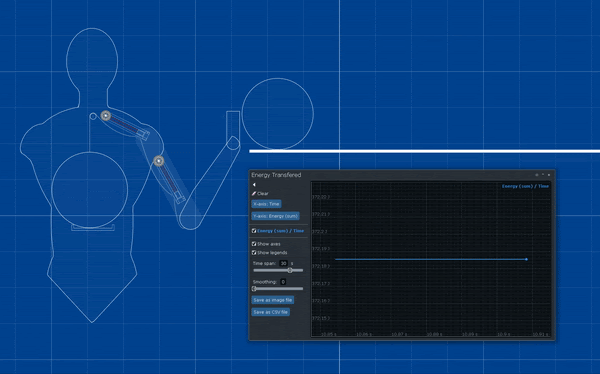
Notice the second spike of energy as the weight slows. Also, notice how although 2 muscles of equal strength are working together, you don't get double the output due to them being only slightly out of sync. Synchronicity plays a huge role in force delivery and is not to be underestimated.
That’s pretty much it! Now all we have to do is combine everything we know so far to recreate the famed draw cut.
The Draw-cut
When putting it together, it’s helpful if you think of energy transfer as water inside a container. The direction, speed, and pressure of water within the container are dependent on the container's acceleration and available space. (Pressure increases as water flows into smaller spaces, and water travels in the opposite direction of a bodies acceleration).
He’re we’re going to go through a very basic top-to-bottom cut.
(Pictures are not representative of correct form, just quick sketches to demonstrate principle)
Momentum - We start by “spilling” forwards, allowing our bodies to build up some momentum.
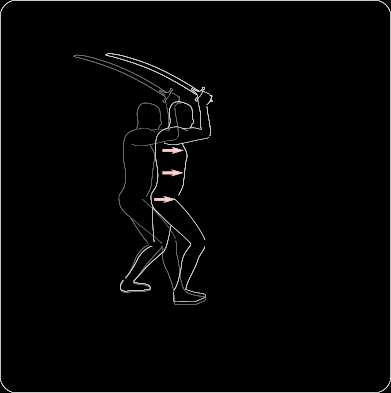
Intiate Energy Transfer - We begin relaxing the body, allowing gravity to take over. This initiates a momentum transfer whilst pulling on our connective tissues, actuating our limbs and reinforcing their movement.
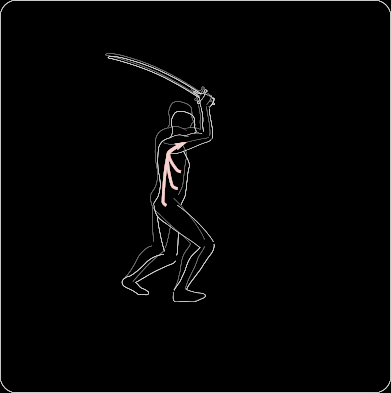
Spilling step / Dhai - As we relax, our hips move backwards relative to our head, as our body accelerates forwards (go backwards to go forwards), driving “heavily” towards the target. This happens with very little effort, using gravity to overcome the body’s inertia, much like the frame of the trebuchet. By doing so, we can lunge into our opponent without putting too much pressure on the ground, reducing the chance of slipping.
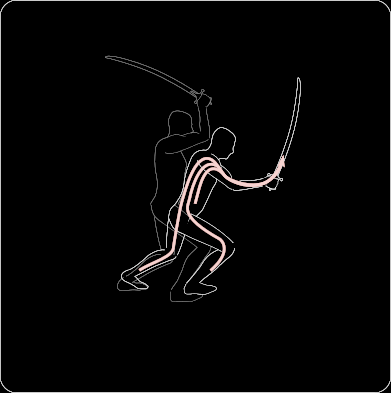
Release - Lastly, energy is released from the body and concentrated in the weapon as our body slows and the weapon makes contact. This contact is relatively soft in comparison to an impact from swinging the weapon, due to the sword having very little momentum of its own before transfer. This reduces shock in the hand and decreases the likelihood of autogenic inhibition limiting our ability to follow through with the strike. With its newly found GPE and kinetic energy, the swords meets the opponent's inertia and travels a short yet extremely energy dense distance, resulting in a strike that feels unexpectedly "heavy", and continues to get heavier as the rest of our body follows through and the swords is drawn through the cut.

All in all, this allows us to not just cut, but drive the cut forwards with our mass, whilst keeping our muscles well below their failure threshold (especially those within the hands). Meaning we have headooom spare to brace against or catch the opponents forward momentum should we need to.
TLDR
To summarise:
We minimise the use of muscle in generating power, due to its tendency to fail under high speeds/loads and to reduce our chances of injury
In place of explosive muscular contraction, we use a combination of fascial tension, GPE and momentum to transfer energy to our limbs/weapons.
We require our body to be as relaxed as possible to facilitate an efficient transfer of momentum unimpeded by asynchronous or counterproductive muscle contractions.
Sound off below
Thank you, can we consider elastic fascia draw/pull reliance rather than gravity, as we are a mobile living system and not machines? Gravity keeps us on the ground but our ability to use the pulling power of connective tissue can actually accelerate down momentum as opposed to only relying on gravity’s impersonal singular force. So in effect our output power is not gravity dependent (nor breath-dependent) as it is centred in our centre core of the body.
Excellent article, informative and very well explained
Probably the most studied and technically descriptive writing of seen on this. It lines up with my own experiences. The down creates the up as they are fond of saying in Chinese classics; I talk to my students about the arms, the forearms and the hands being reluctant to move. Wait for them to be nudged/moved.
I can't imagine how much time and preparation this took. Thanks you!