Structural Strength Vs Muscular Strength ?
- Asante Lawla
- Mar 10, 2024
- 18 min read
Updated: Sep 20, 2024
In the realm of engineering, the term 'strength' refers to a range of mechanical properties, which often diverge from our intuitive understanding of the concept. The conventional image of peak human physical strength —epitomised by olympic lifters with formidable muscles and the capacity to hoist heavy weights overhead—captures just one facet of this multifaceted quality.
However, those who have worked long hours in manual labour environments, have probably had the pleasure of meeting people exhibiting what is often referred to as “farmer strength”. People that seem disproportionately strong for their size. Not just because they're able to out muscle or out work people that are much larger, but they can also apply their strength in all sorts of complicated ways. Whether it be in their technique when using tools or their ability to lift irregular shapes effortlessly. Working on the railway for over 10 years, I've met my fair share of these “farmer strength” types and have been put to shame more than once by people much older than me and with seemingly less muscle.
Although I imagine quite a lot of this comes down to a blend of extensive experience and physical conditioning, I think another major contributing factor is the differing underlying aim in strength development. Training for powerlifting, is all about explosivity and maximal muscle recruitment, whereas with manual labour tasks you generally lean more towards efficiency and just trying not to burn out before the end of your shift. Because of this, movements tend to be comparatively quite relaxed, with a greater focus on minimising unnecessary tension rather than maximising it.
Similarly, in traditional arts when we talk about loading the body in extreme ways, whether it be wearing armour, striking and most definitely when doing some form of grappling. We also prioritise being relaxed and developing what is often described as "structural strength". Characterised by the ability to relax into powerful postures and apply force effortlessly.
In this article I aim to explore the hows and whys of structural strength, and how it differs from muscular strength, to hopefully generate more discussion and understanding in this area of training.
What is Structure ?
So first up, what even is a structure and how could we possibly "relax" into a stronger one ? Isn't that some sort of contradiction, surely your muscles are stronger when they contract ? Well yes, but also no.
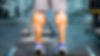
For example, we know that the calves are extremely powerful muscles that produce most of their force when the ankle is plantar flexed to about 80 degrees. Yet when we’re squatting a heavy weight, we don't do so starting on our tip toes with our calves fully contracted. We instinctively "relax" these muscles enough so that the feet aflat on the ground, allowing us to better distribute load across our feet. This in turn allows us to reserve our powerful calf muscles and use them only when it’s most effective.
In short, this is the fundamental difference between structural strength and muscular strength. Muscular strength is a measure of the amount of force your muscles are capable of producing, whereas structural strength is how your posture affects your ability to use those muscles efficiently.
With that said, let’s dive a little more into how structures work from an engineering standpoint so we can get an even better understanding, before we look at the body in more detail.
Rigid Structures
When we talk about creating a structure in engineering, the objective is to increase the distribution of load in a uniform and balanced manner to prevent stress concentrations.
For example, a very simple way of improving a structure is to widen its base to distribute the load over a larger area, just like being flat footed as opposed to being on our tip toes when squatting. But Increasing the distribution alone is not enough. We also want that load to be ‘uniformly’ distributed. That way we can get as much material helping to support the load as possible. A perfect example of this is a pyramid. The taller a building becomes the more load the lower structure has to bear. In a pyramid each layer gets lighter as the building gets taller, resulting in better load distribution.

Equilateral triangles around a square base ensure load is distributed more evenly across the structure.
If we want to build an even taller structure however, although we could just continue to widen the base, this would drastically increase costs, weight, the required strength of the materials, as well as taking up far too much space (unless of course, you happen to be an ancient civilisation). Instead we remove any unnecessary material to reduce weight, then create opposed triangles, aka the classic crosshatch, to distribute load across the structure evenly. The key here is ensuring force vectors are parallel and angles are equal but opposing so that forces essentially balance one another in creating a zero sum.
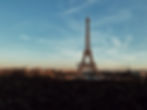
The Eiffel Tower bearing its elegantly engineered structure
Finally to ensure a structure is solid, we use joining and or fastening systems to hold everything in place. We essentially store energy in materials by stretching and or compressing them within their elasticity range –the extent to which a material can deform and return to its original shape – then lock them in place. As they strain to return to their original shape, that restoring force can be used to bind components together or reinforce them, making them better at resisting deformation. Like a stretched and taut guitar string as opposed to a slack one, or the strength of pressurised can opposed to an empty one (but more on this later).
This is where our first more nuanced problem may rear its head. If we decide on using fastening systems, it's paramount that the tension relationships at joints are also well balanced. Whether you use simple rope or nuts and bolts, if these joints aren't properly balanced, you'll end up creating stress concentrations. Areas or individual fastenings bearing more load than their fair share. Imagine what would happen if all but one of the fastenings in a structure was loose? Well that solitary tight fastening will now have to compensate and bear everything that the others aren’t, which on failure can trigger a domino effect of structural failures, as each next best fastening has to bear even more than the last. (Not unlike autogenic inhibition we spoke about in the last article)
This is partly why nuts and bolts are so ubiquitous in engineering. Aside from them being able to withstand an insane amount of load, we can use torque wrenches to accurately measure the amount of tension we put in each bolt. This allows us to not only ensure that bolts are tensioned accordingly but also balance the pretension within the bolt, with the predicted load, ensuring it’s well within the theoretical elasticity range.

Ok, Up to this point everything is fairly straightforward, balanced load, balanced angles, balanced tension. But what about when that predicted load becomes a bit more unpredictable ? Sure we could just increase the size of the bolts to withstand that wider range, but at some point this approach hits a wall of inefficiency. As the structure gets heavier, more problems arise as requirements increase and practicality goes out the window.
The fundamental limitation of rigid designs like the ones we’ve discussed, is they can only stretch or compress so far before failure. They have a fairly narrow, ideal operating range. When dealing with broader loading patterns you have to either manually change the pretension in fastenings or periodically swap them out entirely due to cyclic loading causing fatigue in the materials. This kind of seasonal maintenance is actually quite common. For example on the railway bolts have to be slackened and retightened to allow for expansion during the summer months.
When the predicted load can fluctuate more widely however, engineers switch to using a different kind of design altogether with ‘softer elements' for greater adaptability. While rigid structures are good at dealing with short-lived high frequency vibrations, they struggle with longer lower frequencies like those associated with very high wind speeds and earthquakes which induce much broader variations in loading.
Flexible Structures
So we need a structure to still be practical, but be able to withstand a much wider range of forces…?
What if we could get a structure to distribute load, in a uniform manner, whilst dynamically adjusting to match the load on the fly? Well this idea is at the heart of many ingenious flexible designs.
These flexible designs typically fall into two categories based on their primary mechanisms: those that utilise tension through expansion, and those that leverage compression.
Tension
A classic example of flexible design are suspension bridges. They’re lightweight yet almost unproportionally strong and able to; adapt to powerful cross winds, evenly distribute complicated loads and dampen vibrations caused by earthquakes.
Unlike previous structures we've discussed, which used fixed rigid connections. This design uses the increased elasticity range of twisted steel cables, combined with flexible joints to automatically adjust to varying conditions.
Without going into too much detail, forces acting upon the bridge, whether it be from the weight of passing vehicles or wind, cause a network of interconnected cables to stretch, store and release tension. This allows them to not only distribute complicated loads efficiently across the network, but also transfer or dissipate load in ways rigid materials just can’t, due to their narrow elasticity range. This network in turn compresses the towers/pillars and decking which enhances the structures rigidity, broadening the range and type of load the bridge can accommodate. Even the weight and shape of the bridge itself, is meticulously calculated to utilise gravity in pre tensioning the entire system.

Not leaving anything to chance, these benefits are carried over to the foundations. This entire structure is sat atop specialised ‘isolation bearings’ which; absorb vibrations, allow for thermal expansion and dampen movement. Not too dissimilar to suspension systems found in vehicles.
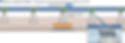
Pressurisation
Speaking of suspension, this brings us quite neatly onto the other very common type of dynamic system, which is the adaptive suspension systems found in buses and trains. Not in quite the same category as buildings and bridges I know, but still very relevant to the discussion of flexible design. Trust me It will make a bit more sense later.
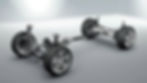
The average car uses shock absorbers and springs which compress in response to load. The force they exert is directly linked to the amount they are compressed. This means the vehicle height and spring length in a normal 5 door car can absorb just enough load for the weight of 1- 5 people + luggage. But what happens when we apply the same system to larger vehicles, carrying anywhere from 1 to 100+ people, distributed at random?
An uncontrolled varying suspension height can start becoming problematic quite quickly. Especially if everyone decides to sit on one side of the bus, where certain conditions may cause excessive rolling and dangerous bouncing. This wide range of expected load benefits from a different approach altogether.
This is where adaptive suspension systems come into play. Busses and trains use specialised air suspension systems equipped with sensors and an ECU, constantly exchanging information. These form a feedback loop that automatically adjusts pressure within rubber cylinders, allowing the vehicle to subtly match load on the system and maintain a desired ride height, regardless of load distribution.
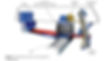
One of the cooler applications of this tech is seeing luxury vehicles achieve an unreal smooth ride and even jump over an obstacle o.0
Human structure
So where does that leave us regarding the human body? Well by now it should be clear that “strength” is highly subjective and the approach to increasing strength really depends on the expected load and type of loading. What is strong in a fairly stable predictable environment, can be very prone to failure in an unstable one. So it shouldn't be any surprise that how we might approach building human strength, could also differ considerably.
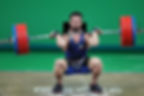
When we look at olympic weightlifting for example, we’re looking at a known quantity of weight, following a predetermined path, exerting entirely predictable loading on the body. Because of this weightlifters can use structures that lean towards rigidity via maximal muscle contraction, in a highly practised and perfected sequence. In fact it's not uncommon to see even the slightest misplacement of posture completely ruin a lift at pro level.
As strong as weightlifters are in the absolute muscular sense, the perfection of their technique and the predictability of the environment are equally important factors to consider when talking about strength and technique.
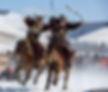
Traditional combat on the other hand, is extremely dynamic by nature. Where the need to dampen, dissipate or even amplify and transfer load plays a significant role. In traditional warfare, there’s controlling the violent vibrations of horse riding in armour, the tumultuous environment of wrestling, and the unknown varying resistance of cutting through material, flesh and bone. Even the very nature of weapon wielding requires a very different approach to the term “strength”. I.e. The underlying mechanics rely far more on the subtle manipulation and transfer of momentum / load via the fingers, than it does on the creation of explosive force in the legs seen in sports like boxing or olympic weight lifting.
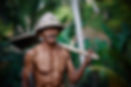
It's not just combat either, using a shovel to dig a formation requires adjusting to varying levels of ground density, without pulling a muscle (something I'm intimately familiar with), and applying force in a biomechanically complicated fashion. Where the ground may be slippery, soft and uneven, whilst resistance can be sudden and unpredictable. Where attempting to use a practised extremely rigid movement, pushing muscles close to failure, is far more than likely to cause injury than success.
This I believe is at the heart of “farmer” or “structural strength”. There's just a certain kind of specificity that goes hand in hand with unpredictable loading and aiming for efficiency over maximal output. A kind of low effort strength where the body has developed well adapted muscular and neuromuscular responses for specific movements. Making it seem unproportionally strong when pitting muscular dexterity against brute strength.
So let's get into it!
Human Structure

A great article from the squat university showing how ideal structure changes depending on where the weight is positioned.
Human bodies have a lot of similarities to the stuff we’ve discussed so far. We have ;
Load bearing bones (beams),
A network of connective tissues (cables)
A pressurised mid section which we can alter at will,
Muscles which can increase or decrease tension in the body (passively or actively)
In terms of creating “structure”, we also care about balancing length tension relationships, and joint angles for good posture.
Where things change however, is in the methods we can use to reinforce / correct this structure. With nuts and bolts it's just a case of using a torque wrench to ensure we have the correct tension in each bolt. Whereas in corrective exercise, changing length tension relationships requires a slightly more nuanced approach.
We can tighten up the muscles that are underperforming via exercise, and relax those that are too tight via stretching or SMR. But as we mentioned earlier, changing tension does not automatically give you a better structure. In fact, there's a fairly common misconception about back pain where people believe that strengthening their back will automatically help to alleviate the pain. Although this may be true in some cases, if your postural habits are acting with gravity to put more load on your spine than your abdomen, greater tension in the lower back is bound to return. In fact, adding more tension to the back could actually make the problem worse by increasing stress concentration in the area.
This is why backsliding (no pun intended) is so common with injuries. We seem to get better when weight + gravity forces our body to move in certain ways, then slowly get worse again once we stop training, or worse yet reinforce bad habits by working around them. It’s not just the habits either, the fundamental biomechanical processes we use to create tension in the muscles like alpha-gamma coactivation where we “manually” tension our structure. Is very different to relying more on the automatic myotatic reflex to form structural habits.
So rather than approach posture from a tension based perspective first and immediately begin to tighten up the slack, or vice versa. Traditional arts like Shastar Vidiya, Taijiquan and various others with “internal” aspects, first try to entirely relax the body, allowing us to isolate for and focus purely on how our body’s weight and shape work with gravity to distribute load. It's a subtle but important difference.
We can overcome muscle imbalances with all kinds of strategies just as architects can design all sorts of shapes and engineers will always find ways of reinforcing them. But to specifically look at our relationship with and awareness of gravity, points your structure in a specific direction. Particularly if we’re aiming to maximise our operating range for traditional warfare.
Traditional Structure / Posture
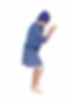
Gurudev Nidar Singh demonstrating a basic posture
Soft body
In all physical tasks building muscle is important for; endurance, strength, mass, bone density and protection. But in addition to this, Shastar Vidiya puts a great deal of focus on developing “soft” or flexible structures and mechanics aiming for adaptability above all.
In regular conversation there's really only two ways we talk about structure. It’s either we’re upright and braced, or slouched. Rarely do people speak about the neutral spot in between. When we go to the gym we're told to brace your core, and lift your chest before doing a rep. A very conscious and deliberate process to ready the body for general sport. It ensures your structure is well supported to protect your joints, with a great deal of load being distributed through hard* contracted muscle tissue and bone. But this posture is very different to one you might adopt if you had to wear armour for months on end. Where actively lifting the chest and tightening the core is a sure fire way to tire yourself out. Traditional postures aim to balance load distribution through bone and “soft” connective tissues equally. Actively avoiding any excessive tension that may sap energy over longer periods. To be honest, even if you wore a 20kg weighted vest for a day, you’d probably find yourself doing some of this naturally. Like;
Relaxing the shoulders and back to reduce strain on the neck
Shifting your centre of mass slightly forwards, and posteriorly rotating the hips to relieve pressure on the lower spine.
Relaxing the core, allowing the weight of the torso and vest to pressurise the intra abdominal cavity for extra support.
But as with all techniques, there are levels to it. Progressing in this kind of training requires systematically and intentionally removing tension from our posture with an aim to feel and adjust load distribution intuitively. The methods for achieving this vary, depending on pre-existing impairments, habits or cultural backgrounds. But one thing that stands as a hallmark of good fighting posture, is the ability to almost “ sit” into your frame.
Much like our suspension bridge, when our shape and weight work together with gravity in just the right manner, our entire structure all the way down to our feet can distribute load efficiently enough to reduce effort on our part to a minimum. This gives rise to a sensation that feels like your body is quite literally “sitting” on itself, in the same way that you might sit on a chair. Being aware of your body’s weight, but not actively playing a part in bearing it.
It doesn't take much to imagine how practical and advantageous this would be if your job involved a great deal of standing or sitting still for that matter. That's without mentioning the muscular and neuromuscular resources that are freed up, and can now be dedicated to more pressing tasks, like not dying. So what about power generation ?
The Subtle Body
With loading taken care of, what about power and all this talk of subtle bodies and visualisation we hear so much about in traditional arts? The answer will always be somewhat subjective depending on who you ask, but I'll share my understanding regardless, based on current science about muscle function.
As much as scientists know about the body, how we perform complex natural movement still isn't quite fully understood. The closest we have to this is the muscle synergy hypothesis and how fascia may play a large role in it (more on that in a moment). What we do know however is how sensors in our muscles respond to load, and what role our motor neurons play.
If we’re going to understand power generation then at the very least, we should understand a little about different types of muscle contraction in the body.
Muscle Function
We have alpha motor neurons, carrying signals responsible for voluntary muscle contraction (extrafusal fibres). Then there are gamma motor neurons, responsible for signals relating to sensory fibres (Intrafusal Fibres). Technically both are muscle fibres of course, but just to make it a little more intuitive to read, that's how I'll refer to them from here onwards.
Have you ever picked up something that was much lighter than expected and almost pulled your back out ? Or have you been to the doctor and experienced a knee jerk reaction, after being attacked with a hammer ? Both are functions of different underlying processes for coordination.
Alpha Gamma Coactivation

Image from https://cpb-us-e1.wpmucdn.com/sites.psu.edu/dist/6/25760/files/2015/04/Muscle-Stretch-Reflex.pdf
Alpha Gamma Coactivation is a process involving conscious communication between our brain, muscle fibres and sensory fibres. Our Brain sends a contraction signal to our muscle fibres via alpha neurons, which contract based on current or expected load, but in doing so inadvertently slacken our sensory fibres, robbing us of information about that load. This means a secondary signal has to be sent via gamma neurons to tell our sensory fibres to remain tight during the movement. It's a bit like telling someone to do something, then sending a second person to check on them and repeating this process 1000s of times a second. It’s no surprise that alpha gamma coactivation signals fatigue over time and are slower than our reflexes. When the expected load doesn't match the actual load, that's when our pulled back scenario occurs.
The Myotatic Reflex

Rather than communicating with the brain, the gamma loop describes signals sent between the spine, muscle fibres and sensory fibres directly. When our muscles stretch, either via relaxing or experiencing some form of load, they mechanically stretch our sensory fibres increasing their signal firing rate. These signals are sent to the spine and directly back to the muscle fibres to subtly or drastically adjust tone. In this scenario the contraction amount is directly linked to load from sensors, creating a kind of balance, where errors due to slackening are less of an issue. It also means any forces that cause our muscles to stretch suddenly, like our doctor's hammer, can cause an immediate automatic contraction in the corresponding muscles, known as the myotatic / stretch reflex
Ideomotor Reflex

Beyond the more direct systems for coordination, there are Ideomotor reflexes. This refers to a physiological phenomenon where a thought or idea causes a subconscious, involuntary physical response. In essence sometimes merely thinking about an action or experiencing certain feelings can cause our muscles to contract without conscious effort or input. A typical example might be wincing or reacting to someone else's pain as if it's your own. This and the stretch reflex are often linked to another subconscious concept called muscle memory. It describes our ability to perform increasingly complex movement without conscious effort due to repeated practice and experience. I’m pretty sure anyone who’s engaged in sports or played instruments at a high level probably has a good idea of what this feels like already.
The Motor Synergy Hypothesis

Finally, overseeing all of this, is a hypothesis that states, rather than trying to control and monitor every single muscle within the body individually, the brain creates modular synergies or groups that allow simple control of complex actions. Modern studies suggest that this is augmented by the fascia which acts not only as a structural component of the body, but a way of transferring large amounts of information across sensory fibres and bone to improve coordination further. Although this hypothesis still has a way to go before being set in stone, it seems fairly intuitive at least on a mechanical level. We know that muscles can respond to stretching directly, without brain input. And we know that the fascia stretches between muscle and bone and can transmit forces across the body.
Power
As mentioned in this article and explored in depth in the last, power in the battlefield context relies on precise coordination of the body, in order to transfer momentum to our weapons. When we go to the gym and lift weights, we engage in voluntary alpha gamma coactivation, where we know how much we’re about to lift, exactly how we’re going to load the body during, and over time learn to perfect this sequence for power. But It should be no surprise, that although this training is of course beneficial, it's unlikely to develop the adaptive kind of strength and power we've been discussing thus far.
If we really want to harness our bodies ability to adapt in real time to complex load, and transfer/amplify momentum efficiently. We need to work on our faster, subconscious coordination abilities as much as our conscious ones. So it follows that the training methods to achieve this would benefit from a different approach to conventional weight training altogether.
A great example of this, which has a lot of historical relevance in various traditional arts including Shastar Vidiya is club wielding.
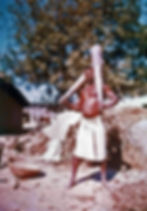
Indian wrestler club wielding https://en.wikipedia.org/wiki/Gada_%28mace%29#/media/File:Indian_wrestler_exercising._1973.JPG
It's one thing to “lift” weights, with the aim of increasing load on the body. It's another thing entirely to “wield” a club and study the passive yet complex stretching it induces within the body.
In Shastar Vidiya, we focus not on the weight of the club, which is kept below a certain threshold to minimise isolated muscle activation, and instead focus on relaxing further and deeper into each movement. Allowing our muscles to stretch, and fascia to carry more and more information across the network, augmenting our coordination. We then focus on improving our awareness and understanding of the subtle manipulation our body engages in, via breathing and adjustments to our posture that highlight these effects. All whilst developing ever deeper holistic motor skills for the use of momentum, weaponry and the body synergistically.
Going beyond this, between developing our subconscious reflexes and deeply ingrained motor skills, lie a set of skills that, though subjective, are just as real as any other in coordinating our body. Simple visualisations and exercises that allow us to tauten our structure by directing our thoughts or asking the body to move in very specific ways. In doing so we can change our neuromuscular response. This borderline subconscious subtle manipulation of the body, in time graduates to more dexterity in application. Just as it's common to hear experienced musicians and dancers describe moving purely based on feeling. as with all things ancient, there are load bearing warrior variants of this phenomena. Where we seek to trigger synchronised and well structured movements through feeling as opposed to thought and reacting to danger or need in place of sound. In a battle that may encompass innumerable combatants, constant life or death decision making and highly dynamic engagement, it stands to reason that the training to achieve this would probably diverge considerably from what we envisage when we think about "strength" training.
It's outside the scope of the article to go into more depth (the irony isn't lost on me, but this article is already long enough lol) but I hope this has at very least piqued your interest in the topic and given you some more things to discuss and explore in your training. Sound off below and let me know your thoughts!